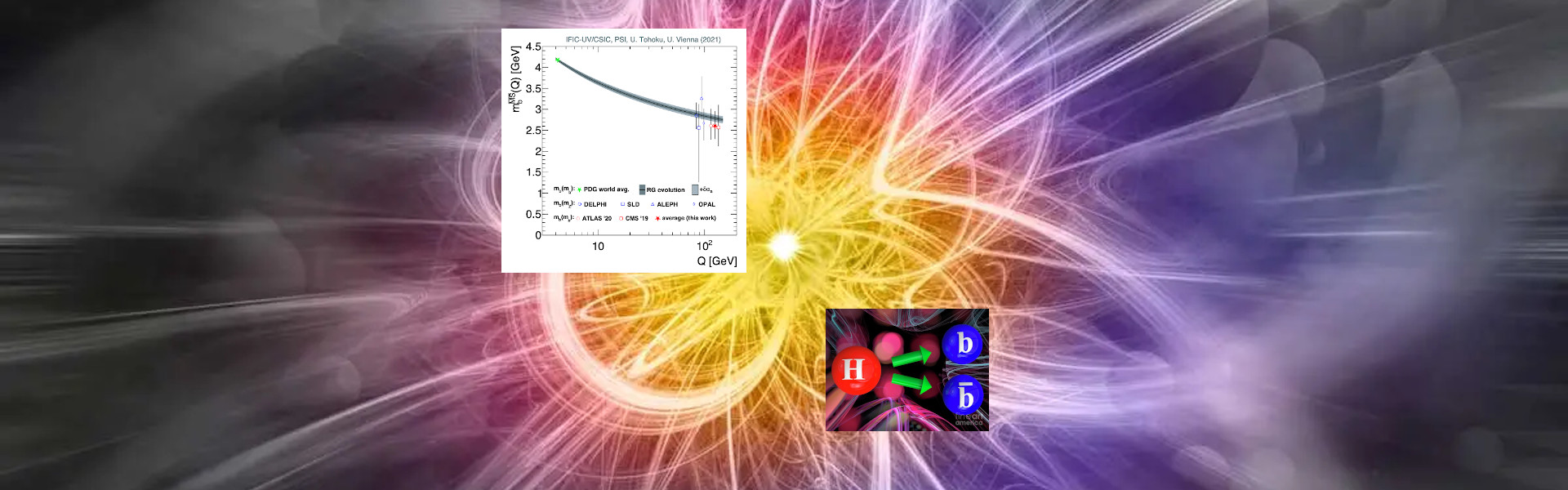
Why are the masses of elementary particles not invariant?
IFIC researchers, in collaboration with researchers from the Universities of Vienna and Tohoku and the Paul Scherrer Institute, measure for the first time the mass of the bottom quark from its interactions with the Higgs boson and confirm, as predicted by theory, that it is not an invariant quantity.

In physics, the mass and weight of an object represent two essentially different concepts. The mass of an object is a measure of the amount of matter it contains, unlike weight, which is a measure of the gravitational force to which it is subjected. According to Newton’s laws, that gravitational force or weight is proportional to mass, so the two concepts are often used interchangeably, albeit inappropriately, in colloquial language.
An object at sea level is slightly less heavy at the top of Everest because it is farther away from the center of the Earth, enough so that the intensity of gravity is slightly weaker. On the surface of the Moon, where the intensity of the gravitational force is six times weaker than the one on Earth, its weight is correspondingly six times less. Its mass, on the other hand, is an invariant quantity, characteristic of the object and independent of its location. To be precise, instead of asking «how much do I weigh?», we should always ask the question «how much mass do I have?».
In the microscopic world something similar happens. Although the force of gravity is not usually relevant among elementary particles, they are subject to other fundamental forces such as the strong, weak and electromagnetic forces, and the intensity of all these forces is not always the same, but depends largely on the energy at which the elementary particles accelerate and collide with each other.
The extraordinary thing in this case is that due to the quantum laws governing the microcosm, the masses of the elementary particles also cease to be an intrinsically immutable quantity. That variability, however, is not arbitrary. Although the theory called the Standard Model cannot predict the value of the masses of elementary particles or the intensity of the fundamental forces, it does predict with great precision how they change as a function of energy. That is, by measuring the masses and intensities of the forces in one experiment at a reference energy, we can predict what their values will be in another experiment where the particles interact at a different energy.
This variability is determined by the quantum fluctuations of the vacuum allowed by the theory. These fluctuations generate from the vacuum virtual particles that appear and disappear affecting the mass and the intensity of the forces depending on the distance, or equivalently energy, at which we observe the particle. The so-called quarks should, according to the Standard Model, become lighter and lighter as we perform experiments at higher energies. In contrast, the Higgs boson and leptons, like electrons and muons, would be more massive.
Proving that the masses of elemental particles change depending of the energy of the experiment is, in the first place, a prove of its quantum behavior. Measuring this change with high precision allows, on the one hand, testing of the Standard Model predictions at the limit and, on the other hand, detecting the possibility of existence of new particles or forces in case that the data diverges from the expected evolution.
The first proof of the variability of a quark’s mass was performed using data from LEP, the predecesor of the Large Hadron Collider (LHC) at CERN, by researchers from IFIC, joint center from CSIC at University of Valencia. This work, in which both theorist and experimentalist researchers from IFIC cooperate closely, proved that the bottom quark’s mass was 40% lighter in LEP comparison with other experiments performed at lower energies.
“Quantum vacuum fluctuations are responsible for the fact that the masses of elementary particles are not invariant.”
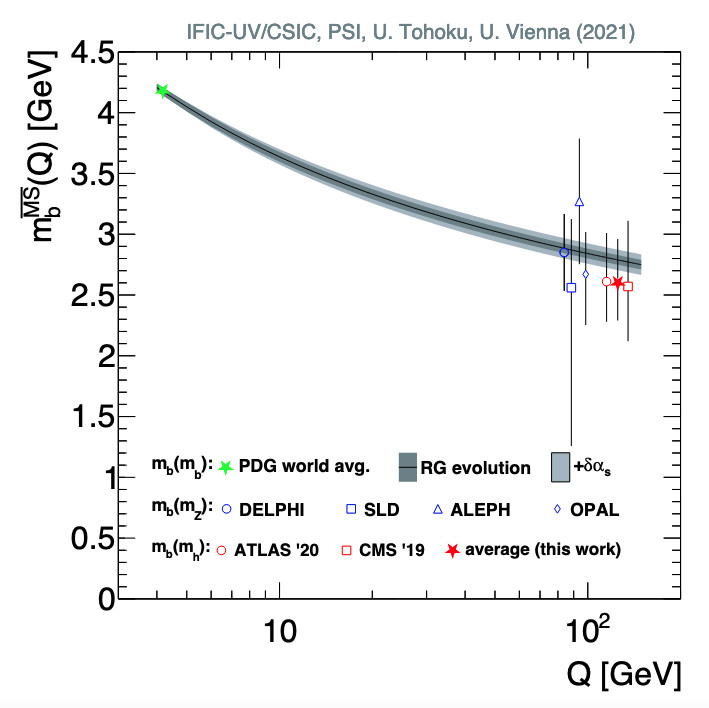
The discovery of the Higgs boson and the observation of its disintegration into pairs of bottom quark and its antiparticle in the LHC allows a completely new measurement of the bottom quark’s mass at a higher energy. To perform this, researchers from IFIC, in collaboration with researchers from the Universities of Vienna and Tohoku and the Paul Scherzer Institute close to Zürich, have used the most advanced theoretical predictions know to date.
This work have been publish in Physical Review Letters, where researches have presented a new measurement of the bottom quark’s mass with a precision of 14%. The value obtained is compatible with the predictions of the Standard Model and rules out the invariance hypothesis with a statistical significance of almost 7 standard deviations. In particle physics, at least 5 standard deviations are required for a result to be considered consolidated. The results presented are the first measurement of the bottom quark mass at the energy scale equivalent to the Higgs boson mass, which is 40 times higher than the quark mass itself, and further strengthen the evidence for its evolution originating from quantum processes occurring at the microscopic scale.
The work also includes a prospective study on the improvements that could be obtained in the new data acquisition phase of the LHC that is about to begin, as well as data from future accelerators such as the International Linear Collider (ILC).
Written by G. Rodrigo & Translated by J. P. Márquez & P. Martínez
Reference
J. Aparisi, J. Fuster, A. Hoang, A. Irles, C. Lepenik, G. Rodrigo, M. Spira, S. Tairafune, M. Vos, H. Yamamoto and R. Yonamine,
mb at mH: the running bottom quark mass and the Higgs boson, Phys. Rev. Lett. 128, 122001. DOI: https://doi.org/10.1103/PhysRevLett.128.122001
Un comentario